Abstract
In this paper the economical, ecological and social sustainability of nuclear power reactors is investigated.
Firstly, the historical records of U.S. power plants overnight costs show a more then tenfold increase during the periode in which almost the entire U.S. reactor fleet was build (throughout the 70’s, 80’s and partly in the 90’s of the last century). It is shown that this is not sustainable in a capitalistic society. Thus it is concluded that this is the only plausible reason (and not pressure from environmentalists) for the cancellation of ca. 100 plants in the U.S. In addition it is speculated upon the costs for nuclear fuel, given the data from official sources. It is shown, that the historically low prices for uranium between ca. 1985 to 2005 can not be maintained, not even in the near future. The actual fuel costs over the last decade support this result.
Secondly, given the lower ore grade of the remaining raw uranium ressources, also the ecological superiority of nuclear fuel is challenged.
Lastly, by presenting some important aspects of this type of electricty generation which are usually not present in the public debate, the social non-sustainability of nuclear power is questioned, too.
1 Introduction
Global warming due to man made greenhouse gas (GHG) emissions is a fact as is the out of this following change of the global climate. Humanity faces tremendous suffering if the emission of greenhouse gasses isn’t stopped as soon as possible [1].
In the „Working Group III Contribution to the Fifth Assessment Report of the Intergovernmental Panel on Climate Change“ [2] state Sims et al. [3] that the transportation sector alone „was [in 2010] responsible for approximately 23 % of total energy-related CO2 emissions“ and Lucon et al. [4] state a figure of 19 % for the buildings sector.
Without giving references one can easily find many individual measures, technical and behavioural related, to reduce the emission of greenhouse gasses within these sectors; e.g. can the material related emission of greenhouse gasses in buildings be reduced to just 30 percent by the use of modified cement [5].
Probably the greatest potential to reduce GHG emissions is to electrify the many human related activities. In contrast to the other two main GHG-emitting sectors of human society — industry and agriculture — can the processes determining the former two sectors relatively easy be transformed into electricty driven operations. The ever more presence of electrical cars is a shining example of this development. However, also the building sector has tremendous GHG reduction potential because during the many years of use of a building, three to almost up to ten times as much energy is used compared to its construction phase [6, 7, 8] and actions like heating or cooling space and water can easily be performed by electrical devices.
But looking at how electrical energy is produced, the situation does not improve with the widespread technology in use as of today. E.g. in the U.S. 2/3 of the electricity was in 2015 produced by GHG emitting technologies (coal, gas or oil) [9]1. Thus electricity production was in 2015 responsible for ca. 30 % of the U.S. total GHG emissions. The same amount as the whole U.S. transportation sector [10]2.
Thus it has to be concluded that technologies have to be employed that generate electricity by non-greenhouse gas emitting processes.
The vast majority of electricity producing machines utilize the inversion of the electromagnetical motion discovered by Michael Faraday [11]: a rotating spool in a static magnetic field (or the other way around) leads to the generation of an electrical current. Usually these spools are driven by turbines which themself are driven by a hot gass, usually watervapor. An exception to this are solar cells, because these use a different physical process to produce electricity. Also windturbines or hydroelectric power stations don’t employ hot gass. There the rotation of the spool is (more or less) directly driven by the moving wind or water.
However, the water must be heated to evaporate and be able to drive a turbine. This is achieved by burning coal, oil or gas or by using the heat released in nuclear reactions. The three former directly emit greenhouse gasses when burned. Hence, such technologies for electricity generation should be replaced as soon as possible. On account of this, one often can hear or read, that more nuclear reactors should be build to meet the demand for electricity since this technology is considered to be „emission-free“. Probably the publicly best known proponent of nuclear energy as solution for climate change is James Lovelock [12] and another typical example for nuclear energy support in the public debate may be the Washington Post’s opinion from 2017-04-01 which commented on „Westinghouse“3 going out of business under the headline „A bankruptcy that’s bad news for climate policy“ [13].
A scientific paper that provides many of the exemplary talking points of nuclear power advocates may be „Why nuclear energy is sustainable and has to be part of the energy mix“ by Brook et al. [14].
According to the Nuclear Energy Institute were „[…] 11 percent of the world’s electricity production in 2014 […]“ provided by nuclear power plants [15].4
However, electricity is so called secondary energy converted from primary energy. Coal, oil or nuclear fuel can be considered as primary energy carriers which are transformed into secondary energy e.g. in power plants (electricity) or oil refineries (gasoline). If Nuclear Energy shall contribute substantially to the reduction of GHG emission, it must be measured against the world primary energy consumption since GHG emitting resources are used for e.g. the heating of houses (e.g. in form of coal) or transportation (in form of oil). The world primary energy consumption can be found for example in the BP Statistical Review of World Energy [17] and is shown in fig. 1.
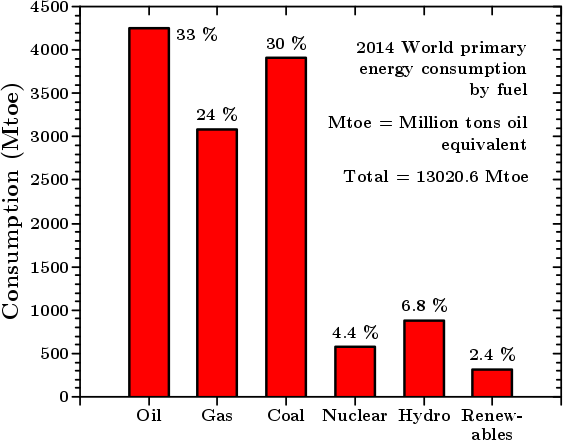
Fig. 1: 2014 world primary energy consumption. Data taken from table „Primary energy: Consumption by fuel“, p. 41 in [17]. The International Energy Agency presents in [16] very similar numbers.
Taking the lower average electrical capacity of nowadays nuclear reactors into account actually 1150 to 1750 new reactors have to be build.
In a capitalist society this means that more then 1000 times non-state actors have to take the financial risks of building a nuclear power plant.
With these numbers in mind, this paper is meant to refute the position that nuclear energy is a solution to climate change mainly on economic grounds. I try to show that nuclear energy is by no means an „[…] affordable, economically viable […] energy service […]“ as has been called for at the United Nations World Summit on Sustainable Development in Johannesburg fifteen years ago [20].
I outline how the some of the economic aspects of nuclear energy have developed in the past and what that may mean for the future. The latter especially under the angle if this technology shall be used to substitute e.g. coal plants. This whole topic is very involved, due to the complexity of the underlying technologies. Hence I concentrate on the economic sustainability of nuclear power in this paper. Some ecologic and social issues are briefly covered at the end of this report.
2 Method
To research the topic of the sustainibility of nuclear power plants mainly publicly available technical and statistical reports and some scientific papers were evaluated. The figures given there were compiled, compared and/or visualized for analysis and the conclusions drawn from this analysis are presented in this paper.5
However, this paper should not be seen as a literature overview.
The biggest limitation of this analysis is that it is U.S. centric due to the chosen/availibility of first hand documents. However, this is not to be seen as a major disadvantage or may not limit the conclusions due to the following reasons. Firstly, almost one fourth of all operating nuclear reactors can be found in the United States. Approximately one third of the electricity worldwide generated by nuclear power plants is produced by these [18]. Thus it can be concluded that the U.S. situation can reasonably be used as a model for the proposal to employ more nuclear power.
Secondly, the United States can be seen as the model country for a capitalistic system. That means that as many enterprises as possible should be inititated, build, maintained, operated etc. by private actors. At least all profitable enterprises. This is the basic assumption under which the economical conclusions below are made. If this assumption, that nuclear power has to function under capitalistic conditions, is not true (e.g. because nuclear power is wanted for political, defense, prestige, research, medical or many other reasons) then the below stated conclusions may still be true, but irrelevant.
Thirdly, the situation in France (the country with the second largest nuclear reactor fleet) is pretty similar [23].
Footnotes
- 1. Table 1.1. Net Generation by Energy Source: Total (All Sectors), 2007-March 2017 ↩
- 2. Table ES-6: U.S. Greenhouse Gas Emissions Allocated to Economic Sectors (MMT CO2 Eq.) ↩
- 3. One of the largest nuclear power reactor producers. ↩
- 4. Or 10.6 percent according to the International Energy Agency [16]. ↩
- 5. All data within the context of nuclear reactors presented here is just for commercial nuclear power plants. Though, due to the lack of data, a few plants had to be omitted by Koomey and Hultman from their analysis, tables A-1a and A-1b in [21] show extensive data for almost all U.S. nuclear power plants that eventually started operation. This includes the dates when construction started and commercial operation actually began. However, the docket numbers are missing. In Table 1 in [22] all applied for nuclear units up to March 1978 are listed, including their docket numbers. However, due to the publishing date of this report, finishing times of a lot of the units were estimated, this includes units that eventually got cancelled. Appendix A in [18] presents some data for the nuclear reactors still operating (incl. the dates when the construction permit was issued and commercial operation started). Appendix D in the same source presents data for cancelled nuclear power reactors. Comparison of these three sources lead to the numbers presented in fig. 4 and fig. 5.
All units that are listed as operating or cancelled/withdrawn in any of these three data sets, up to the stated years, are included in fig. 4. Not included are units that were stated as „uncertain“ in [22], Watts Bar unit 2 (docket number 50-391) and the Bellefonte units 1 and 2 (docket numbers 50-438/439) since these three are still under construction [18]. The WPPSS unit 2 (docket number 50-397) in [22] was identified to be „Columbia Generating Station“ in [21] and [18]. ↩
References
- [1] K. Richardson et al., „Synthesis Report from Climate Change – Global Risks, Challenges and Decisions, Copenhagen 2009“, University of Copenhagen, ISBN: 978-87-90655-68-6 ↩
- [2] O. Edenhofer et al., „Climate Change 2014: Mitigation of Climate Change. Contribution of Working Group III to the Fifth Assessment Report of the Intergovernmental Panel on Climate Change“, Cambridge University Press, 2014. ↩
- [3] R. Sims et al., „Transport“ in „Climate Change 2014: Mitigation of Climate Change. Contribution of Working Group III to the Fifth Assessment Report of the Intergovernmental Panel on Climate Change“, pp. 599–670. Cambridge University Press, 2014. ↩
- [4] O. Lucon et al., „Buildings“ in „Climate Change 2014: Mitigation of Climate Change. Contribution of Working Group III to the Fifth Assessment Report of the Intergovernmental Panel on Climate Change“, pp. 671–738. Cambridge University Press, 2014. ↩
- [5] K. Celik et al., „Mechanical properties, durability, and life-cycle assessment of self-consolidating concrete mixtures made with blended portland cements containing fly ash and limestone powder“, Cement and Concrete Composites, vol. 56, pp. 59–72, 2015. ↩
- [6] R. J. Cole and P. C. Kernan, „Life-Cycle Energy Use in Office Buildings“, Building and Environment, vol. 31, no. 4, pp. 307–317, 1996. ↩
- [7] B. N. Winther and A. G. Hestnes, „Solar Versus Green: The Analysis of a Norwegian Row House“, Solar Energy, vol. 66, no. 6, pp. 387–393, 1999. ↩
- [8] O. F. Kofoworola and S. H. Gheewala, „Life cycle energy assessment of a typical office building in Thailand“, Energy and Buildings, vol. 41, pp. 1076–1083, 2009. ↩
- [9] U.S. Energy Information Administration, „Electric Power Monthly with Data for March 2017“, 2017 ↩
- [10] U.S. Environmental Protection Agency, „Inventory of US Greenhouse Gas Emissions and Sinks 1990 – 2015“, 2017 ↩
- [11] M. Faraday, „On some new Electro-Magnetical Motions, and on the Theory of Magnetism“ in chapter „Papers on Electricity from the Quarterly Journal of Science, Philosophical Magazine, &c“ in „Experimental Researches in Electricity 2“, pp. 127–147 Richard and John Edward Taylor, 1844. ↩
- [12] J. Lovelock, „Nuclear power is the only green solution“, The Independent, 2004-05-23 (accessed 2017-07-20). ↩
- [13] Editorial Board of the Washington Post, „A bankruptcy that`s bad news for climate policy“, Washington Post, 2017-04-01 (accessed 2017-07-20). ↩
- [14] B. W. Brook et al., „Why nuclear energy is sustainable and has to be part of the energy mix“, Sustainable Materials and Technologies, vol. 1-2, pp. 8–16, 2014. ↩
- [15] Nuclear Energy Institute, „World Statistics Nuclear Energy Around the World“, 2017 ↩
- [16] International Energy Agency, „Key world energy statistics“, 2016 ↩ ↩
- [17] „BP Statistical Review of World Energy June 2016“ ↩ ↩
- [18] U.S. Nuclear Regulatory Commission, „Information Digest 2016-2017, NUREG-1350“, vol. 28, 2016. ↩ ↩ ↩ ↩ ↩
- [19] S. Ansolabehere et al., „The Future of Nuclear Power“, Massachusetts Institute of Technology, 2003. ↩
- [20] Team of Authors, „Plan of Implementation of the World Summit on Sustainable Development“, United Nations World Summit on Sustainable Development, 2002. ↩
- [21] J. Koomey and N. E. Hultman, „Electronic Annex for A reactor-level analysis of busbar costs for US nuclear plants, 1970–2005“, Energy Policy, vol. 35, pp. 5630–5642, 2007. ↩ ↩
- [22] F. A. Heddleson, „Summary Data for U.S. Commercial Nuclear Power Plants in the United States – ORNL/NUREG/NSIC-141“, U.S. Nuclear Regulatory Comission – Office of Nuclear Regulatory Research, tech. rep., 1978. ↩ ↩ ↩
- [23] A. Grubler, „The costs of the French nuclear scale-up: A case of negative learning by doing“, Energy Policy, vol. 38, pp. 5174–5188, 2010. ↩
Leave a Reply